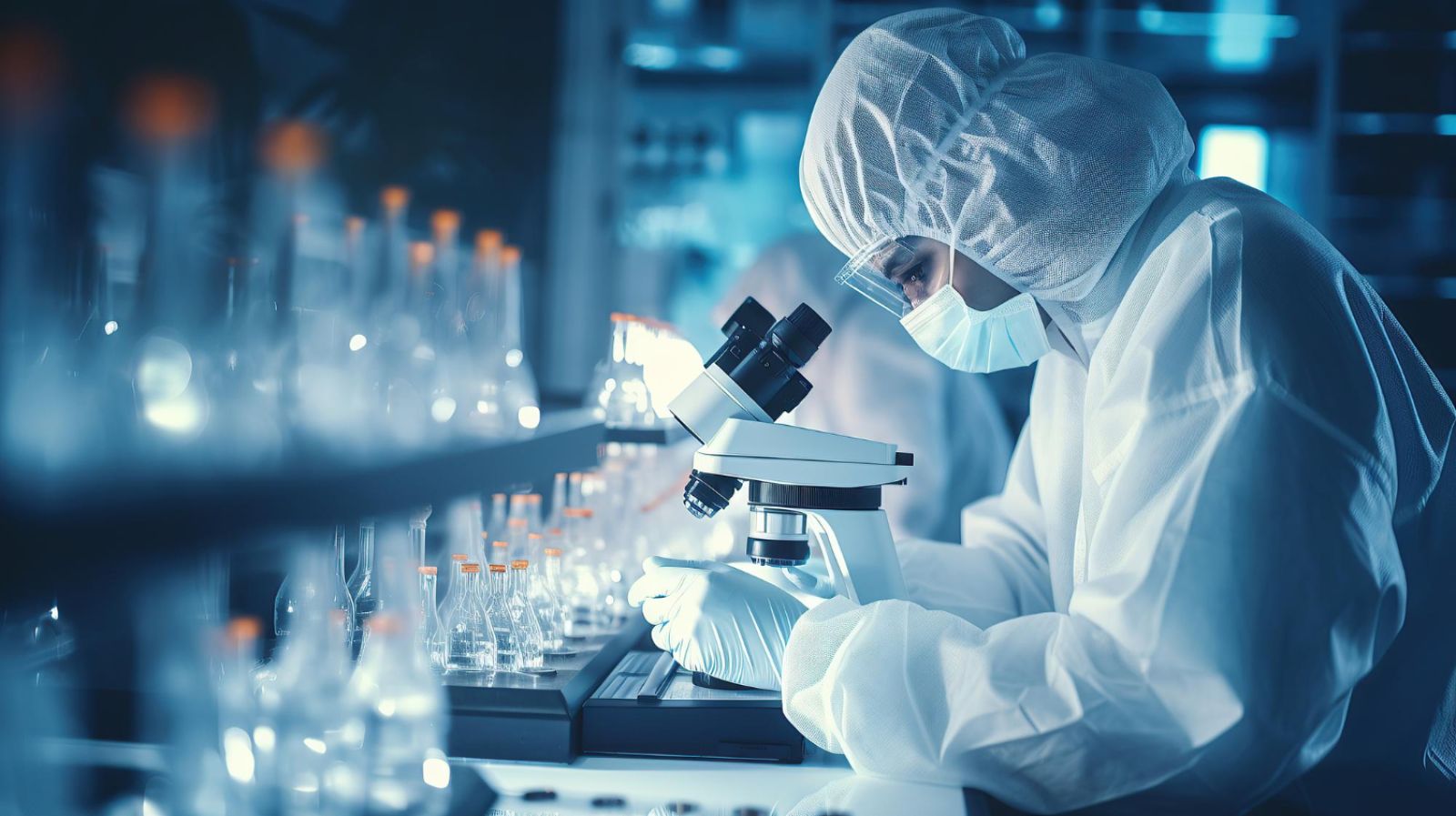
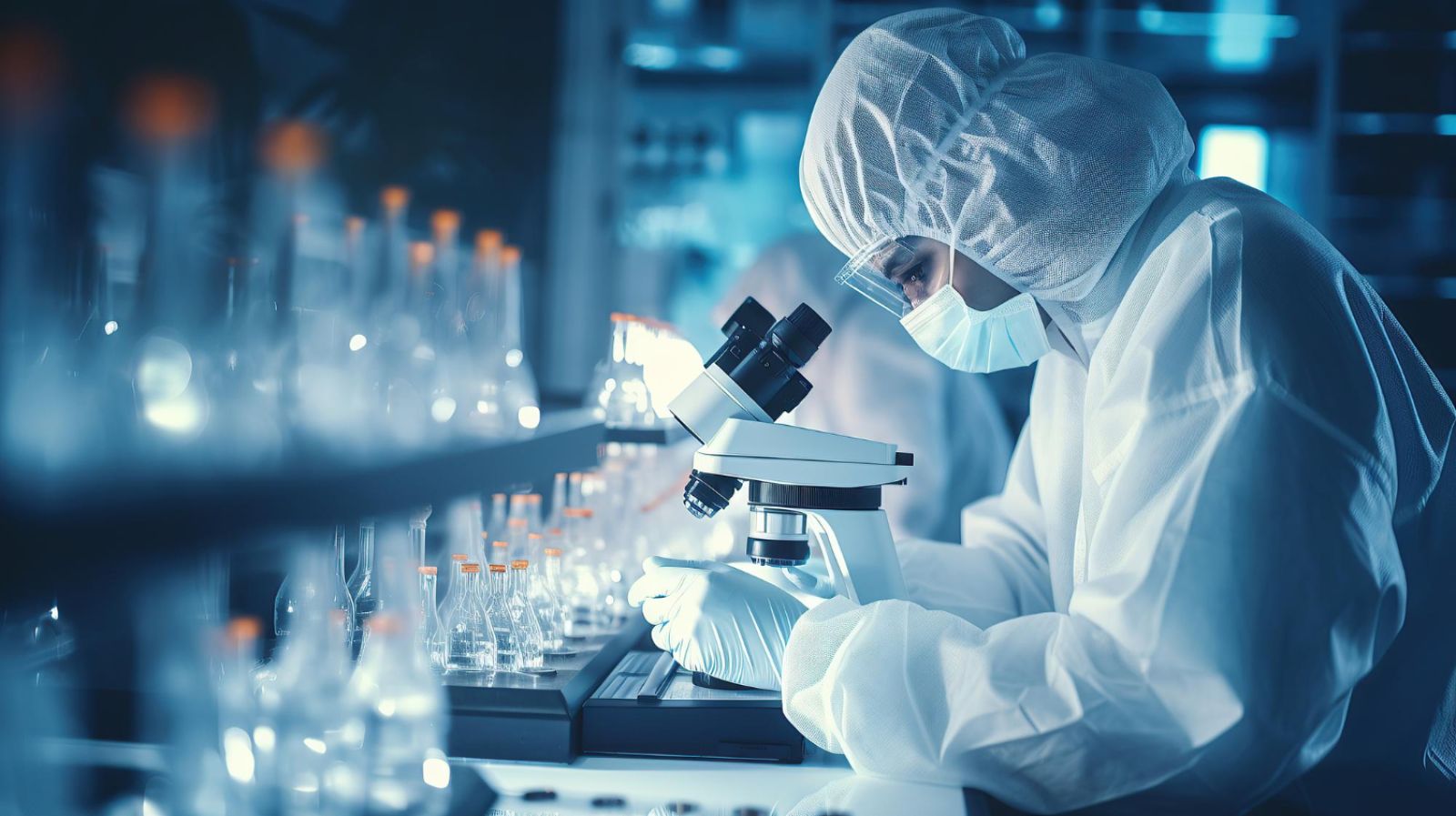
In the 21st century, we have witnessed a staggering number of major infectious disease outbreaks: SARS in 2003, H1N1 swine flu in 2009, MERS in 2012, Ebola in 2014, Zika in 2015, Madascar plague in 2017, Nipah in 2018, and, of course, the COVID pandemic. Consequently, the vaccine industry emerged as a dark horse within the pharmaceutical sector—or more accurately, a dark cow, as the Latin term “vacca” suggests. In 2017, total infectious disease vaccine sales were projected to grow from $25 billion in 2013 to about $35 billion by 2020.[1] However, due to COVID-19 in 2020, the vaccine industry rapidly grew to a whopping $55.44 billion and is forecasted to grow from $61.04 billion in 2021 to $125.49 billion in 2028.[2] This increased demand for vaccines paved the way for the emergence of several new technologies, that would otherwise take years or even decades to develop and approve.
Messenger RNA vaccines – new era in immunization
The onset of the COVID-19 global pandemic presented a singular opportunity to usher in a novel vaccine type: messenger RNA vaccines. Unlike traditional vaccines (inactivated, live-attenuated and subunit), which introduce either a weakened or dead pathogen or a part of it, mRNA vaccines utilize the host’s own cells to produce the immunoreactive protein. As stated by Katalin Karikó, the 2023 Nobel Prize winner for her discoveries concerning nucleoside base modifications that enabled the development of effective mRNA vaccines against COVID-19, “The cell was producing plentiful amounts of protein upon instruction: it was like magic!” [3] mRNA-based vaccines provide good immune response, are safer than live-attenuated vaccines and are considerably easier, faster and cheaper to manufacture. However, there is space for enhancement – a 5 billion Project Next Gen is founded to improve the current COVID vaccine.[4][5]
Future potential of mRNA vaccines
Several potential avenues exist to enhance mRNA vaccines. One such area for improvement involves addressing their short shelf life and specific storage requirements. Unlike traditional vaccines, which can remain viable for up to three years, mRNA vaccines have a maximum shelf life of only six months when stored in ultra-cold conditions. Another potential improvement lies in optimizing the delivery method of mRNA by using Tiba Biotech’s polymer nanoparticle technology instead of lipid nanoparticles. The current delivery method, lipid nanoparticles (LNPs) are suspected to cause reactogenicity. Therefore, incorporating nanoparticles into mRNA technology has the dual benefit of improving thermostability and mitigating adverse effects of the vaccine. [5],[6] An additional challenge associated with the vaccine pertains to the necessity for receiving multiple doses or booster shots. Several strategies exist to adress this issue, with one option being the utilization of self-amplifying circular RNA.
When employing this technology, immunity persists for a duration comparable to that of live-attenuated vaccines, yet without the risks inherent in live-attenuated vaccines. One of the self-replicating mRNA vaccines (Gennova Biopharmaceuticals) has already been approved for emergency use in some countries. [5].Regrettably, a significant obstacle facing mRNA-based vaccines is a considerable level of hesitancy, which appears to stem from similar concerns to those held about GMO products – a fear associated with anything containing “DNA.” [7] As of 2024 only 80% of adults in the United States have been fully vaccinated according to CDC. [8]
RSV vaccines
The surge in interest surrounding mRNA-based COVID vaccines has additionally propelled the development and accelerated approval of other pharmaceuticals. In 2023, a total of 55 new therapeutics obtained approval, representing the second-highest count recorded in the past 30 years. Notably, among these approvals were six vaccines, with particular excitement surrounding those targeting Respiratory Syncytial Virus (RSV). RSV poses significant danger for infants and young adults.The initial unsuccessful attempt to create an RSV vaccine in the 1960s resulted in two fatalities, and more than half a century later, in 2023, the first RSV vaccine, Arexvy(GSK), was approved, followed by the second vaccine, Abrysvo(Pfizer). Both of these vaccines were made possible by the discovery of the crystallographic structure of the F subunit protein. Quoting John Tregoning (Imperial College London, London, UK) on RSV vaccine impact “Where deployed, these will have a huge impact by reducing the burden of infection, particularly on paediatric units in the winter months.” Both Arexvy and Abrysvo are subunit vaccines, with Arexvy specifically being an adjuvanted vaccine [9][10], but there is also an mRNA RSV vaccine from Moderna (mRNA-1345) on the way, which highlights that mRNA vaccine platform has potential beyond COVID-19. [11]
‘One shot is all it takes’
An intriguing prospect within vaccine development for future observation involves the creation of combined vaccines. This approach involves the formulation of vaccines that integrate multiple antigens to target several infectious diseases simultaneously. For instance, Moderna is currently in the process of developing a single mRNA vaccine designed to confer immunity against COVID-19, influenza, and RSV simultaneously with a singular administration. Similarly, GSK is investigating the utilization of exosomes for immunization purposes, with a single vesicle potentially capable of providing protection against SARS-CoV-2 and other coronaviruses by leveraging Codiak BioSciences’ exosome platform. By presenting multiple antigens in a single shot, the potential for a universal vaccine arises, offering protection against various infectious diseases with just one immunization.
No more needles
There is good news for individuals who fear needles (or for parents of such individuals). Intramuscular injection, which is a remnant from the early days of vaccination, may not be the optimal method for vaccine delivery, and as it turns out, vaccines do not necessarily require injection. Interestingly, one of the earliest immunization methods, variolation, introduced smallpox by scratching an individual’s skin with an infected object. The skin, being not only the body’s largest organ but also containing a majority of immune cells, is an apt site for vaccine delivery. In this case, vaccines would be captured by dendritic cells, which would then deliver the antigens directly to the lymphatic system. An instance of such a delivery system was pioneered by Vaxxas. They have engineered a needle-free, high-density microarray patch (HD-MAP), which, when applied to the skin for around ten seconds, administers the vaccine beneath the surface through thousands of coated microprojections. Delivering antigens to mucosa, for example in the form of a nasal spray, appears to be a viable option as well. However, these vaccines may encounter challenges in obtaining approval due to the specificity of markers for mucosal immunity. One example of a vaccine like this is Bharat Biotech International’s nasal vaccine against SARS-CoV-2 that received emergency use authorization in some countries.
In conclusion, the 21st century has witnessed significant advancements in vaccine development and infectious disease control, driven by unprecedented challenges such as the COVID-19 pandemic. These advancements, ranging from the emergence of mRNA vaccines to innovative delivery methods, underscore the resilience and adaptability of scientific endeavors. As we navigate the complexities of future outbreaks, it is clear that continued collaboration and innovation will be paramount in shaping a future where widespread protection against infectious diseases is both achievable and accessible.